Impact of Diabetes Mellitus on Ventricular Structure, Arterial Stiffness, and Pulsatile Hemodynamics in Heart Failure With Preserved Ejection Fraction
Julio A. Chirinos, MD, PhD; Priyanka Bhattacharya, MD; Anupam Kumar, MD; Elizabeth Proto, BA, HES; Prasad Konda, MD; Patrick Segers, PhD; Scott R. Akers, MD, PhD; Raymond R. Townsend, MD; Payman Zamani, MD, MTR Background-—Heterogeneity in the underlying processes that contribute to heart failure with preserved ejection fraction (HFpEF) is increasingly recognized. Diabetes mellitus is a frequent comorbidity in HFpEF, but its impact on left ventricular and arterial structure and function in HFpEF is unknown. Methods and Results-—We assessed the impact of diabetes mellitus on left ventricular cellular and interstitial hypertrophy (assessed with cardiac magnetic resonance imaging, including T1 mapping pregadolinium and postgadolinium administration), arterial stiffness (assessed with arterial tonometry), and pulsatile arterial hemodynamics (assessed with in-office pressure-flow analyses and 24-hour ambulatory monitoring) among 53 subjects with HFpEF (32 diabetic and 21 nondiabetic subjects). Despite few differences in clinical characteristics, diabetic subjects with HFpEF exhibited a markedly greater left ventricular mass index (78.1 [95% CI, 70.4–85.9] g versus 63.6 [95% CI, 55.8–71.3] g; P=0.0093) and indexed extracellular volume (23.6 [95% CI, 21.2–26.1] mL/m2 versus 16.2 [95% CI, 13.1–19.4] mL/m2 ; P=0.0008). Pronounced aortic stiffening was also observed in the diabetic group (carotid-femoral pulse wave velocity, 11.86 [95% CI, 10.4– 13.1] m/s versus 8.8 [95% CI, 7.5–10.1] m/s; P=0.0027), with an adverse pulsatile hemodynamic profile characterized by increased oscillatory power (315 [95% CI, 258–373] mW versus 190 [95% CI, 144–236] mW; P=0.0007), aortic characteristic impedance (0.154 [95% CI, 0.124–0.183] mm Hg/mL per second versus 0.096 [95% CI, 0.072–0.121] mm Hg/mL per second; P=0.0024), and forward (59.5[95% CI, 52.8–66.1] mm Hg versus 40.1 [95% CI, 31.6–48.6] mm Hg; P=0.0010) and backward (19.6 [95% CI, 16.2–22.9] mm Hg versus 14.1 [95% CI, 10.9–17.3] mm Hg; P=0.0169) wave amplitude. Abnormal pulsatile hemodynamics were also evident in 24-hour ambulatory monitoring, despite the absence of significant differences in 24-hour systolic blood pressure between the groups. Conclusions-—Diabetes mellitus is a key determinant of left ventricular remodeling, arterial stiffness, adverse pulsatile hemodynamics, and ventricular-arterial interactions in HFpEF. Clinical Trial Registration-—URL: https://www.clinicaltrials.gov. Unique identifier: NCT01516346. (J Am Heart Assoc. 2019;8: e011457. DOI: 10.1161/JAHA.118.011457.) Key Words: arterial stiffness • diabetes mellitus • hemodynamics • heart failure with preserved ejection fraction • left ventricular hypertrophy • magnetic resonance imaging • myocardial fibrosis The burden of heart failure (HF) has markedly increased over the past several years. Approximately half of HF is secondary to HF with preserved ejection fraction (HFpEF), which is anticipated to represent an even larger proportion of the total burden of HF as the population ages.1 Multiple therapies that provide clinical benefit in HF with reduced EF are available. However, proven pharmacologic interventions to improve outcomes in HFpEF are currently lacking. Patients with HFpEF have a substantial burden of comorbidities that may contribute to its pathophysiological characteristics and may impact its prognosis and response to therapy. From the Division of Cardiovascular Medicine, Hospital of the University of Pennsylvania, Philadelphia, PA (J.A.C., P.B., A.K., P.K., R.R.T., P.Z.); Division of Cardiovascular Medicine, University of Pennsylvania Perelman School of Medicine, Philadelphia, PA (J.A.C., A.K., E.P., R.R.T., P.Z.); Biofluid, Tissue and Solid Mechanics for Medical Applications, Ghent University, Ghent, Belgium (P.S.); and Department of Radiology, Corporal Michael J. Crescenz Veterans Affairs Medical Center, Philadelphia, PA (J.A.C., S.R.A.). Correspondence to: Julio A. Chirinos, MD, PhD, South Tower, Room 11-138, Perelman Center for Advanced Medicine, 3400 Civic Center Blvd, Philadelphia, PA 19104. E-mail: julio.chirinos@uphs.upenn.edu Received November 7, 2018; accepted January 22, 2019. ª 2019 The Authors. Published on behalf of the American Heart Association, Inc., by Wiley. This is an open access article under the terms of the Creative Commons Attribution-NonCommercial License, which permits use, distribution and reproduction in any medium, provided the original work is properly cited and is not used for commercial purposes. DOI: 10.1161/JAHA.118.011457 Journal of the American Heart Association 1 ORIGINAL RESEARCH Downloaded from http://ahajournals.org by on February 17, 2019 Heterogeneity in the underlying processes that contribute to HFpEF is increasingly recognized. Diabetes mellitus is a key risk factor for HF, and a frequent comorbidity in patients with HFpEF, which is associated with poor outcomes in this patient population.2 Increased aortic stiffness, arterial dysfunction, and cardiac remodeling have been reported in diabetic patients in the general population, in patients with chronic kidney disease, and in patients with left ventricular (LV) systolic dysfunction.3–10 However, no data are available about the influence of diabetes mellitus on LV remodeling/fibrosis, arterial stiffness, pulsatile arterial hemodynamics, and ventricular-vascular interactions in HFpEF. HFpEF is often accompanied by diabetes mellitus, obesity, and various other comorbidities that impact LV remodeling and arterial function,3,11,12 but is associated with structural cardiovascular abnormalities in its own right.13 Furthermore, HFpEF differs from HF with reduced EF in terms of ventricular remodeling and ventricular-arterial coupling patterns.14–18 Detailed hemodynamic and cardiac phenotyping can provide important insights into the pathophysiological characteristics of LV remodeling, fibrosis, and abnormal ventricular-arterial interactions, which contribute to the pathophysiological characteristics of HFpEF.19,20 In this study, we aimed to assess the impact of diabetes mellitus on LV hypertrophy, diffuse myocardial fibrosis, large artery stiffness, ventricular-vascular interactions, and in-office and 24-hour pulsatile arterial hemodynamics in HFpEF. Methods We analyzed data from a previous phase 2 trial that was designed to assess the effect of isosorbide dinitrate, isosorbide dinitrate plus hydralazine, or placebo on pulsatile hemodynamics and LV remodeling in HFpEF.21 The current study used only baseline (preintervention) phenotypic data. The data, analytic methods, and study materials are not publicly available for purposes of reproducing the results or replicating the procedures. Such data may be made available to other researchers for collaborative research, through the establishment of appropriate data sharing agreements and regulatory approvals. Study Population Inclusion criteria for the trial included symptomatic HFpEF (LVEF >50%), in addition to at least one of the following: (1) prior hospitalization for decompensated HF; (2) short-term treatment for HF requiring intravenous diuretics or hemofiltration; (3) echocardiographic evidence for elevated filling pressures22; (4) long-term treatment with a loop diuretic for control of symptoms; (5) or an elevated NT-proBNP (N-terminal pro-B-type natriuretic peptide). Subjects needed to be on stable medical therapy for the past month. Exclusion criteria included any rhythm other than sinus with native conduction; noncardiac conditions that significantly limit exercise (orthopedic or neuromuscular); known hypertrophic, infiltrative, or inflammatory cardiomyopathy; pericardial disease; significant pulmonary disease; primary pulmonary arteriopathy; acute coronary syndrome or coronary revascularization within the past 60 days; clinically significant perfusion defects on stress imaging without subsequent revascularization; significant valvular disease (eg, moderate or greater mitral regurgitation or aortic stenosis); uncontrolled hypertension (systolic blood pressure [SBP] >180 mm Hg or diastolic blood pressure [DBP] >100 mm Hg); prior reduced LVEF 6.5%. Enrollment was not performed in regards to diabetic status and, therefore, there was no matching procedure to enroll diabetic versus nondiabetic subjects in the parent trial. Clinical Perspective What Is New? • Compared with nondiabetic subjects with heart failure with preserved ejection fraction, diabetic subjects with heart failure with preserved ejection fraction exhibit more left ventricular hypertrophy, myocardial extracellular volume expansion, pronounced aortic stiffening, and an adverse pulsatile hemodynamic profile, characterized by increased oscillatory power, aortic characteristic impedance, and forward and backward (reflected) wave amplitude. • Abnormal pulsatile hemodynamics in the diabetic subgroup with heart failure with preserved ejection fraction are also evident in 24-hour ambulatory monitoring, despite the absence of significant differences in 24-hour systolic blood pressure between the groups. What Are the Clinical Implications? • Heterogeneity in the underlying processes that contribute to heart failure with preserved ejection fraction is increasingly recognized. • Diabetes mellitus is responsible for important differences in relevant underlying phenotypes in this patient population. DOI: 10.1161/JAHA.118.011457 Journal of the American Heart Association 2 Diabetes Mellitus in HFpEF Chirinos et al ORIGINAL RESEARCH Downloaded from http://ahajournals.org by on February 17, 2019 Echocardiography and Arterial Tonometry Echocardiography was performed using a Vivid e9 or Vivid I machine (General Electric, Fairfield, CT). Echocardiographic parameters of diastolic function were quantified according to American Society of Echocardiography guidelines by a trained cardiologist (P.Z.)22; each metric was quantified in triplicate, with average values presented. Volumetric flow was quantified using pulse-wave Doppler measurements from the LV outflow tract in the 5-chamber view (Figure 1) and the LV outflow tract cross-sectional area measured in the parasternal longaxis view, providing a time-resolved aortic flow waveform. Applanation tonometry was performed at the carotid, radial, and femoral arteries using a high-fidelity tonometer (Millar Instruments, Houston, TX), with a single-lead ECG recording. The ECG complex of the ECG was used as a fiducial point. Surface measurements were obtained from the sternal notch to the site of interrogation at the carotid and femoral arteries to compute carotid-femoral pulse wave velocity (CFPWV), a measure of large artery stiffness. Specifically, the path length was computed as the distance from the sternal notch to the femoral interrogation site minus the distance from the sternal notch to the carotid interrogation site. Radial tonometric waveforms were calibrated using the brachial SBP and DBP, obtained using a validated oscillometric device (Omron HEM-705CP [Omron Corp, Kyoto, Japan] or Accutorr Plus [Datascope Corp, Paramus, NJ]), assuming no brachial-to-radial amplification. Mean arterial pressure was computed as the mean pressure from the radial pressure waveform. Carotid tonometry, calibrated using mean arterial pressure and DBP, was used to obtain a central pressure waveform (Figure 1). Tonometric signals were processed using Sphygmocor software (AtCor Medical, Australia). Central Pulsatile Arterial Hemodynamics The pressure-flow pair was used to perform pulsatile hemodynamic analyses (Figure 2). Pressure-flow analyses were performed by a technician and overread by a cardiologist with expertise in pressure-flow analyses (J.A.C.). Custom-designed software programmed in Matlab (R2014b; MathWorks, Natick, MA) was used to assess aortic pressure-flow relations, as described previously in detail.19,23 In brief, after alignment of signal-averaged central pressure and flow waveforms, we computed aortic input impedance as the ratio of central pressure/flow in the frequency domain. Aortic root characteristic impedance was quantified as the average of impedance modulus at higher frequencies (Figure 2). Wave separation analysis was performed to obtain the amplitude of the forward and backward pressure waves. Reflection magnitude was defined as the ratio of backward/forward pressure. Ambulatory Central BP Monitoring and Pulse Wave Analysis Ambulatory BP monitoring over 24 hours was performed using the Mobilograph device (IEM, Stolberg, Germany), Figure 1. Measurement and signal averaging of central pressure and flow using arterial tonometry and Doppler echocardiography. DOI: 10.1161/JAHA.118.011457 Journal of the American Heart Association 3 Diabetes Mellitus in HFpEF Chirinos et al ORIGINAL RESEARCH Downloaded from http://ahajournals.org by on February 17, 2019 which measures brachial and central (aortic) BP, as well as time-resolved waveforms, allowing for ambulatory pulse wave analysis (including wave separation into forward and backward waves, as shown in Figure 3).24–26 We analyzed data over 24 hours, as well as daytime and nighttime indexes separately. Cardiac Magnetic Resonance Imaging: LV Structure and Function Cardiac magnetic resonance imaging (CMR) was performed in 44 of the studied subjects. Participants underwent a CMR examination to assess LV structure and function using a 1.5-T whole body magnetic resonance imaging scanner (Avanto or Espree; Siemens Healthcare, Malvern, PA) equipped with a phased-array cardiac coil. LV volumes and EF were determined using balanced steady-state free-precession cine imaging. Typical parameters were as follows: repetition time=2.6 milliseconds; echo time=1.3 milliseconds; phases=30; slice thickness=8 mm; bandHz/pixel; flip angle=70°; field of view=300 to 340 mm2 ; matrix size=1929192; and parallel imaging factor=2. LV short-axis stack cine images were manually traced at end diastole and end systole using CMR42 software (Circle CVI, Calgary, AB, Canada). All magnetic resonance imaging measurements were performed by a trained technician and overread by a cardiologist with expertise in CMR (J.A.C.). LV mass (LVM) was computed as the difference between epicardial and endocardial volumes, multiplied by myocardial density. LVM was normalized for height in meters raised to the power of 1.7.27 We used a modified look-locker inversion recovery sequence to assess T1 times before and after the IV administration of gadolinium contrast (gadopentetate dimeglumine, 0.15 mmol/ kg or equivalent) in a midventricular short-axis slice.28,29 Scan parameters for modified look-locker inversion recovery were as follows: field of view=340 mm2 ; matrix size=1449192; slice thickness=6 mm; repetition time=2.4 milliseconds; echo time=1.18 milliseconds; flip angle=30°; bandHz/pixel; and parallel imaging factor=2. Myocardial T1 measurements were performed before and at several time points (5, 10, 15, and 20–40 minutes) after gadolinium administration. Modified look-locker inversion recovery was performed with a 5-3-3 schema with 2 inversions (5 TIs (inversion times) after inversion 1, 3 T1 recovery heartbeats, and 3 TIs after inversion 2). All available blood and myocardial T1 measurements were used to compute k (the myocardiumblood partition coefficient) as the slope of the myocardial 1/T1 over the blood 1/T1 change, via linear regression.29 The fraction of myocardial tissue comprised by the extracellular space (extracellular volume [ECV] fraction) equals k9(1hematocrit). Extracellular LV volume was computed as LV wall volume multiplied by ECV. Cellular LV volume was computed as LV wall volume multiplied by (1ECV).30 Subjects enrolled in the trial who demonstrated impaired renal function precluding the administration of gadolinium (estimated glomerular filtration rate
Дата добавления: 2019-03-09; просмотров: 125; Мы поможем в написании вашей работы! |
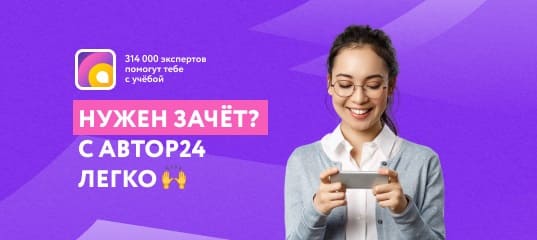
Мы поможем в написании ваших работ!